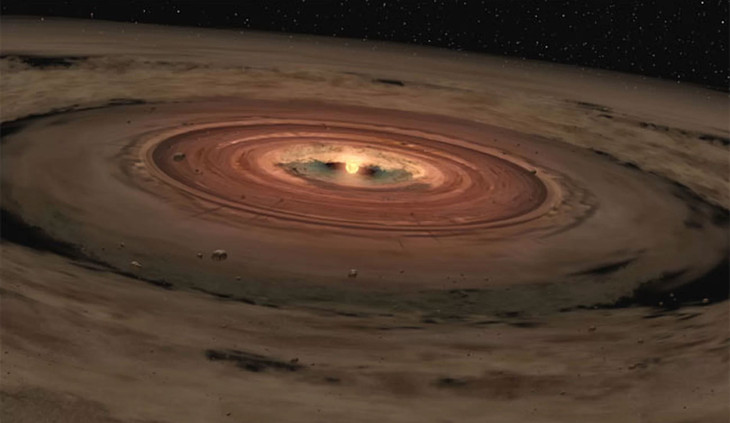
A newly formed star is surrounded by a rotating disk of gas and dust, called a protoplanetary disk. This disk, illustrated here around a brown dwarf, provides the materials for planet formation. IMAGE: NASA/JPL-Caltech
How are solar systems born? Spinning disks and oddball stars and planets help astronomers test theories about the formation of planetary systems
Posted on May 12, 2021UNIVERSITY PARK, Pa. — Many of us remember those school-room models of our Solar System, with tiny wooden planets rotating at the ends of their wires around a bright-orange painted sun. But how accurate is the model? Do the planets really align in a plane, or do their orbits crisscross around the sun at different angles? It turns out the toy isn’t too far off, at least in this one aspect.
Our solar system is actually pretty flat, with most of its planets orbiting within three degrees of the plane of the Earth’s orbit around the sun, called the ecliptic. This flatness extends to the asteroid belt between Mars and Jupiter, though some members of the region of icy objects past Neptune called the Kuiper belt are more extreme, with inclinations up to 30 degrees.
This relative flatness, which is not an unusual feature of solar systems, results from how stars and planetary systems typically form. The process begins with a slowly rotating, roughly spherical cloud of gas and dust, about one light year across. Eventually, a portion of this material collapses toward the center, forming a star, and the spinning cloud begins to flatten into a disk due to its rotation. It’s out of this rotating protoplanetary disk of gas and dust that planets are then spun out, resulting in a relatively flat solar system. Eventually, when most of the gas has settled onto the star or planets or has dissipated, the system is left with a debris disk of planetary leftovers, like our own asteroid-strewn Kuiper belt.
Some astronomers at Penn State study protoplanetary and debris disks to get a better idea of how planetary systems form. But not all stars and planets form in exactly the same manner — and not all planetary systems are flat.
The interdisciplinary nature of astronomy research at Penn State, including at the University’s Center for Exoplanets and Habitable Worlds, allows its scientists to paint a bigger picture of the formation and evolution of planetary systems.
“It’s an exciting time, because so many planets have been discovered in other solar systems, for example by NASA’s Kepler space telescope and Transiting Exoplanet Survey Satellite (TESS), and a lot of them look very different from the planets in our solar system,” said Rebekah Dawson, Shaffer Career Development Professor in Science and assistant professor of astronomy and astrophysics. “So, we have to come up with new ways of thinking about planet formation that can account for the diversity of planets we now know about.”
In addition to studying disks, researchers like Dawson study the exceptions to the norm, unusual stars and planets that could support or make us rethink current theories. Together, these investigations are helping scientists improve our understanding of how and where different kinds of stars and planets form, and what makes a planet habitable.
Catching planetary formation in the act
While some researchers study mature systems and infer aspects of the planet formation process, Assistant Professor of Astronomy and Astrophysics Ian Czekala and ICDS co-hire tries to catch planetary formation in the act.
“I study the protoplanetary disks that surround young stars for the first 10 million years of their lives,” he said. “That may sound like a long time period, but it’s actually very small compared to a star’s total lifetime. Our sun, for example, is about five billion years old; most of the exoplanet systems that people study are a least a billion years old.”
While Kepler and other survey missions have found thousands of mature solar systems, there are fewer nearby protoplanetary systems that easily lend themselves to detailed study. To investigate these early systems, Czekala uses the Atacama Large Millimeter/submillimeter Array (ALMA), one of the most complex astronomical observatories ever built. Located in Chile, ALMA uses a network of high-precision antennas working together to provide a high-resolution look at the universe, using wavelengths of light between the infrared and radio regions of the electromagnetic spectrum.
ALMA can directly detect the gas and dust in protoplanetary disks, which produce very cold thermal emissions (20 to 30 degrees Kelvin, or -400 to -424 degrees Fahrenheit) at millimeter wavelengths. Czekala uses gas in the disk, including carbon monoxide, as a tracer to determine how the disk is rotating. This data provides a glimpse of the disk’s dynamics, building a sort of three-dimensional picture as a function of velocity. Some disks show gaps that might be produced by a planet orbiting in that space.

Example of a protoplanetary disk surrounding young binary stars. The gas and dust in these disks produce cold thermal emissions that can be detected at the millimeter wavelength. IMAGE: ALMA (ESO/NAOJ/NRAO), I. CZEKALA AND G. KENNEDY; NRAO/AUI/NSF, S. DAGNELLO
“What’s interesting is not just that we see the disk rotating, but that we are starting to sense the ways in which the velocity of the field deviates from its expected rotation at a very subtle level,” said Czekala. “It’s like watching a river flow downstream. Sure, you see the bulk flow of the river, but when you look at the eddies and turbulent waves, you can infer that there might be a submerged rock in one area or even a large underwater cavern in another. It’s what lies underneath that gets me really excited.”
Where do planets form?
Dawson in some cases studies debris disks, but she is also very interested in understanding the formation of planets that look nothing like those in our solar system. In particular, she is studying how planetary orbits might have changed in an early solar system, which can tell us how planets came to be where they are today.
“When talking about theories of planet formation, there is some debate about where planets form, even if the processes involved in those different locations may have some similarities,” she said.
Some of Dawson’s research has focused on large gas giant planets called “hot Jupiters” that are similar in mass to our own Jupiter but are found unexpectedly close to their stars. Because of the proximity to their stars, these planets have a surprisingly short orbit of only three or four days.
There are several theories about how hot Jupiters formed. One suggests that they formed where they are currently located, close to their stars. Another theory suggests that they formed farther away, but a disturbance of some sort exhibited a gravitational force that made the hot Jupiter’s orbit extremely elliptical in a way that it passed very close to its star. Eventually, the pull of the star produced tides on the planet that caused its orbit to shrink and become more circular.
“To try to disentangle these possible formation pathways, we sometimes use computer simulations of the process, which leads to other expectations for the properties of hot Jupiters,” said Dawson. “We can then compare observed properties of hot Jupiters based on visible and infrared observations of their stars with the simulations to see if they are consistent with a particular theory. We can also look for planets in the same system as the hot Jupiters for clues to their formation.”

An artist’s depiction of a hot Jupiter gas giant planet, which are similar in mass to our own Jupiter but are found unexpectedly close to their stars. Penn State astronomer Rebekah Dawson studies the history of planetary systems with hot Jupiters by investigating how these large planets came to be so close to their stars. IMAGE: NASA/JPL-CALTECH
If a hot Jupiter forms near its star, for example, it’s plausible that other planets formed nearby that could be observed. But if it is formed through the pathway with a highly elliptical orbit, it’s likely that any other planets between the hot Jupiter and the sun would get ejected or collide with it.
“What we see in the data is that most Jupiters don’t have other planets nearby, but there are a few exceptions,” said Dawson. “I’ve come to believe that none of these theories can explain all of the hot Jupiters that we see. There may be different ways to make a hot Jupiter, and that’s probably true of other types of planets that we see that don’t look like the planets in our solar system.”
Tiny star or giant planet?
Just as the study of unusual planets like hot Jupiters can help us understand fundamental processes, so too can the study of unusual stars. Stars exist across a wide range of masses, the heaviest of which is 150 times the mass of our sun. The lightest stars, known as brown dwarfs, are less than one-tenth the mass of the sun and therefore can be cool and faint enough to look like a gas giant planet.
Kevin Luhman, professor astronomy and astrophysics, has spent much of his career studying how brown dwarfs are like stars and how they are like planets. To determine if they are born more like stars or planets, he is trying to identify the smallest mass at which brown dwarfs exist.
“There are different theories about the formation of stars that make different predictions for the minimum mass at which brown dwarfs exist,” he said. “If you can measure that minimum mass, you can test theories of how stars are born.”
Because they are cool and faint, brown dwarfs can also be challenging to find; the first wasn’t discovered until 1995. However, when they are very young, brown dwarfs are relatively bright — almost as bright as other stars — making them easier to detect.
“We look for newborn brown dwarfs in nebulas of gas and dust that are already known to be giving birth to stars, like the nearby Orion Nebula,” said Luhman. “Much of my work has involved searching these nebulas, using very sensitive telescopes that are able to see them.”

This illustration shows the relative sizes of a hypothetical brown dwarf planetary system compared to our own solar system. Penn State astronmer Kevin Luhman studies how small, cool brown dwarfs are similar to planets and how they are similar to stars, which will provide insight into the process of star and planet formation. IMAGE: NASA/JPL-CALTECH/T. PYLE (SSC)
Luhman has helped identify brown dwarfs as small as five times the mass of Jupiter, which overlaps with the masses of some planets. He hopes that the launch of the James Webb Space Telescope in 2021 will allow astronomers to determine the minimum mass of these unusual stars.
“Brown dwarfs tend to be brightest in the infrared, and James Webb will be the most powerful infrared telescope to date,” he said. “We also hope to answer whether and how often planets can form around brown dwarfs. There’s already good evidence of protoplanetary disks existing around brown dwarfs, meaning they have the building blocks for making planets around them.”
These questions will help inform the bigger picture about planet formation, including whether planets form around any kind of star or only stars like the sun — and, of course, whether it is possible for planets around brown dwarfs, if they exist, to harbor life.
New opportunities
While star and planet formation might be thought of as separate fields of research, the processes involved are all intrinsically linked. Protoplanetary disks not only spawn planets but continuously funnel gas and dust onto the young star. And the very act of planet formation changes the structure of the disk, which may affect the subsequent planets that form in the same disk. It’s somewhat of a chicken and egg scenario, according to Czekala.
In this way, it’s useful to have a variety of researchers using different tactics to study these processes all in one place. The interdisciplinary nature of astronomy research at Penn State, including at the University’s Center for Exoplanets and Habitable Worlds, allows its scientists to paint a bigger picture of the formation and evolution of planetary systems.
“We’re trying to piece together the processes of star and planet formation, but we only glimpse clues here and there, which we need to use to guide us to a holistic theory,” said Czekala. “New observations always lead to a bloom of new theories, but at the end of the day the big picture needs to hang together, including the implications for the formation epoch that we’re studying with the protoplanetary disks. We have a unique opportunity to bring the different communities together.”